Research

Research in the Smith Lab develops advanced methods for catalytic conversion of abundant or waste feedstocks into high-value chemicals.
Discovering catalysts suited for electrification of the chemical industry necessitates exploration of new design spaces and strategies for precise control of electron delivery to break and form bonds. By advancing ultrahigh-throughput experimentation (uHTE) and fundamental analysis of molecular and materials platforms, we will establish new strategies for manipulation of molecules and sustainable chemical synthesis from abundant or waste feedstocks at speeds orders of magnitude faster than traditional experimentation.
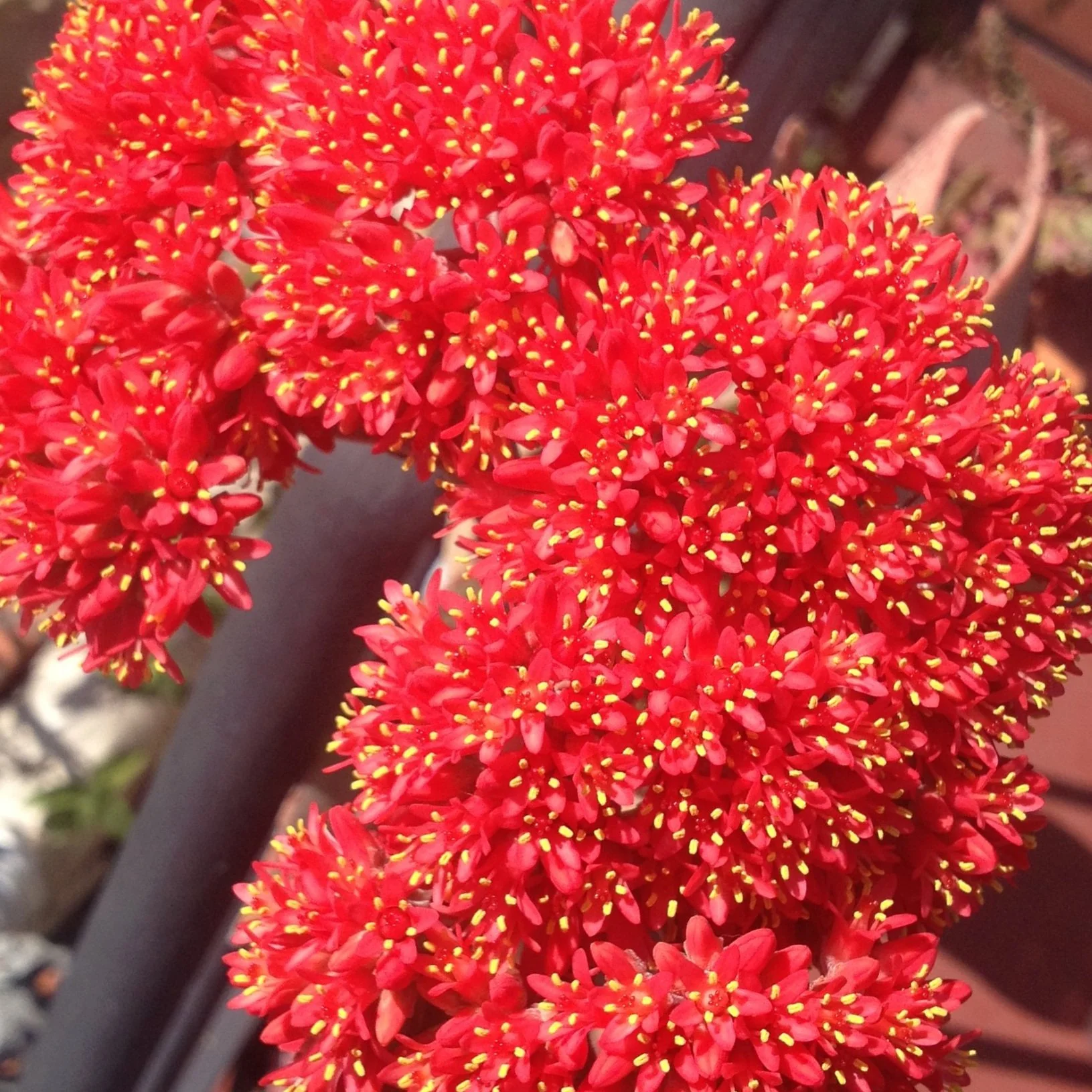
Methane Diversification Through Tandem Photocatalysis
Methane is the simplest C1 hydrocarbon, yet it suffers from limited reactivity that prevents broad synthetic utility. It is a valuable fuel and syngas precursor but is also largely burnt off as waste. Expanding synthetic applications that use methane as a building block is of great interest due to the potential simplicity, efficient atom economy, exploiting an underutilized feedstock, and being a new methylation mechanism. Thus, we will explore frustrated Rh porphyrin metalloradicals for methane activation and C-H bond cleavage in a way to photocatalytically generate methyl radicals. These radicals will then be homogeneously captured by various means, including at boron or nickel species for subsequent reactivity. We will investigate methane oxidation into methanol, methane cross-coupling reactions, and methane carboxylation into acetic acid relying on Si, Ni, and Ir catalysts working in tandem with Rh. These studies will elucidate new methane synthetic chemistry, which will be of great interest to synthesis, medicinal, energy, and commodity communities.
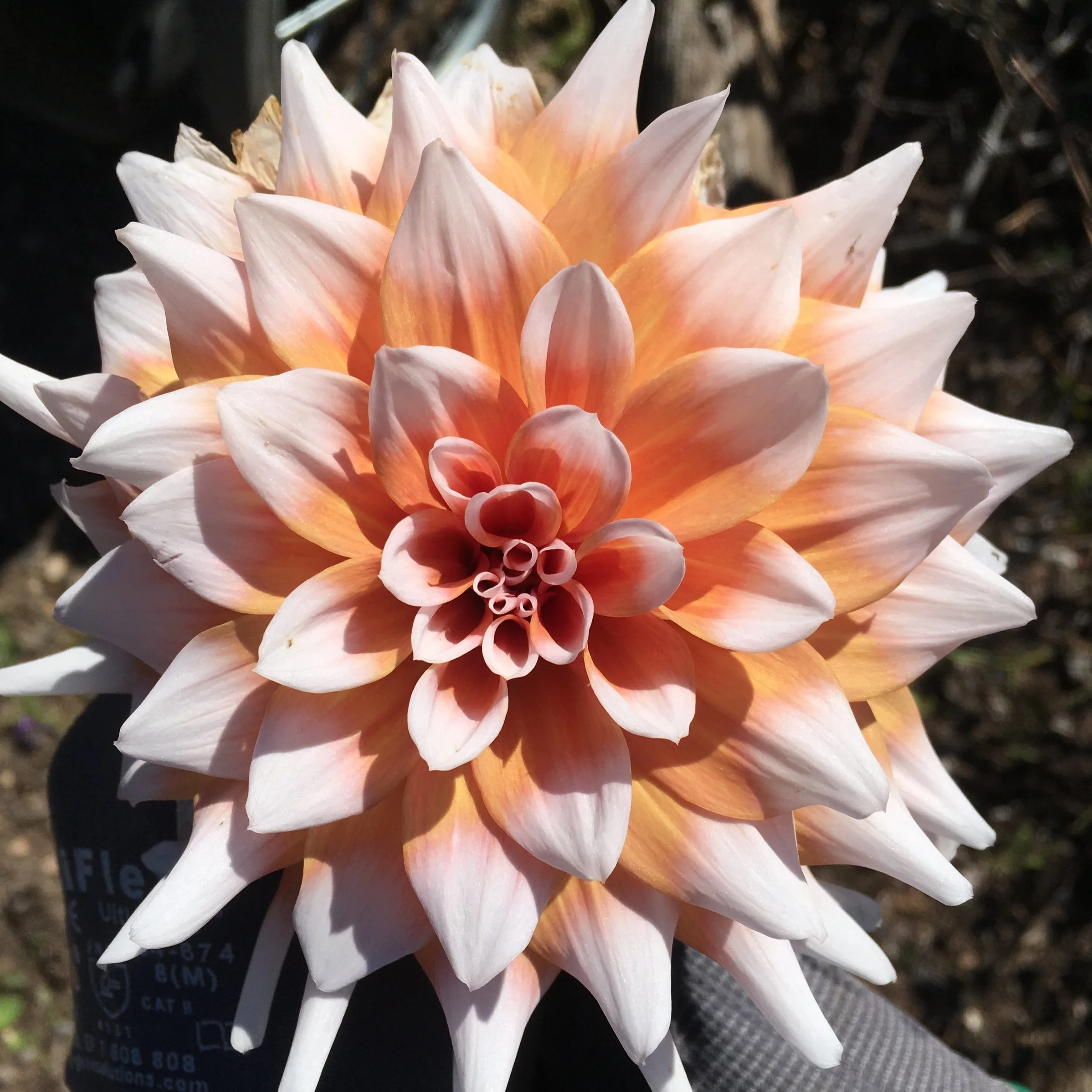
Dual-Input Strategies for Multi-Potential Redox Catalysis
For electrochemical upgrading, most reaction pathways require multi-step electron and proton transfers. Thus, selectivity is highly dependent on the applied potential. Electron/proton delivery can be controlled via ligand design or mediators, but there are limitations to the potentials used to match electron transfer to/from the ligand/mediator with the catalyst/substrate. To circumvent this, we will study dual-input redox control using dynamic potential variation or separate electrocatalysts and photocatalysts that operate in tandem to expose active sites to different redox powers. Mild potentials will be applied to the electrode while introducing photoredox mediators in solution or as intramolecular appendages which operate with significantly higher driving forces for charge transfer. Additionally, dynamically changing the applied potential during catalysis will be explored to combine oxidative bond cleavage and reductive bond formation steps as well as control the nucleophilicity/electrophilicity of adsorbed intermediates. We will create a handle for tuning electron delivery that facilitates challenging product selectivity, such as CO2 reduction to CH2O, CH4 oxidation to CH3OH, C2H6 conversion, and plastic upgrading. This work will teach important lessons about substrate-electrode interactions and provide fundamental insight into how dynamic applied potential methods can control adsorption, oxidation, reduction, and desorption steps for sustainable synthesis.
Ultrafast Catalyst Discovery Tools Using Nanolithography
Materials libraries synthesized by nanolithography are a transformative approach to chemical synthesis capable of accessing millions of nanomaterials with complex element control. This proposal will expand the types of hard/soft nanomaterials compatible with nanolithographic synthesis and interface the megalibraries with molecular cocatalysts and surface ligands. Such modifiers will tailor catalyst electronics, local environments, and tandem reactivity. With megalibraries, we can study massive amounts of cocatalysts/modifiers and their combinations, unveiling valuable lessons for hybrid catalyst design for a broad range of reactions. To screen libraries, we will develop sensors and molecular probes for chemoselective and spatiotemporal detection of product formation or materials restructuring. Molecular probes, microelectrode sensors, and scanning electrochemical techniques with micron resolution will discover unique catalysts worthy of in-depth analysis. Further acceleration of screening can be achieved with automation and machine learning. These generalizable methods will let us study underexplored reactions primed for electrification including hydroformylation, or reduction of phosphate, iron oxide, sulfur dioxide, or carbon monoxide.
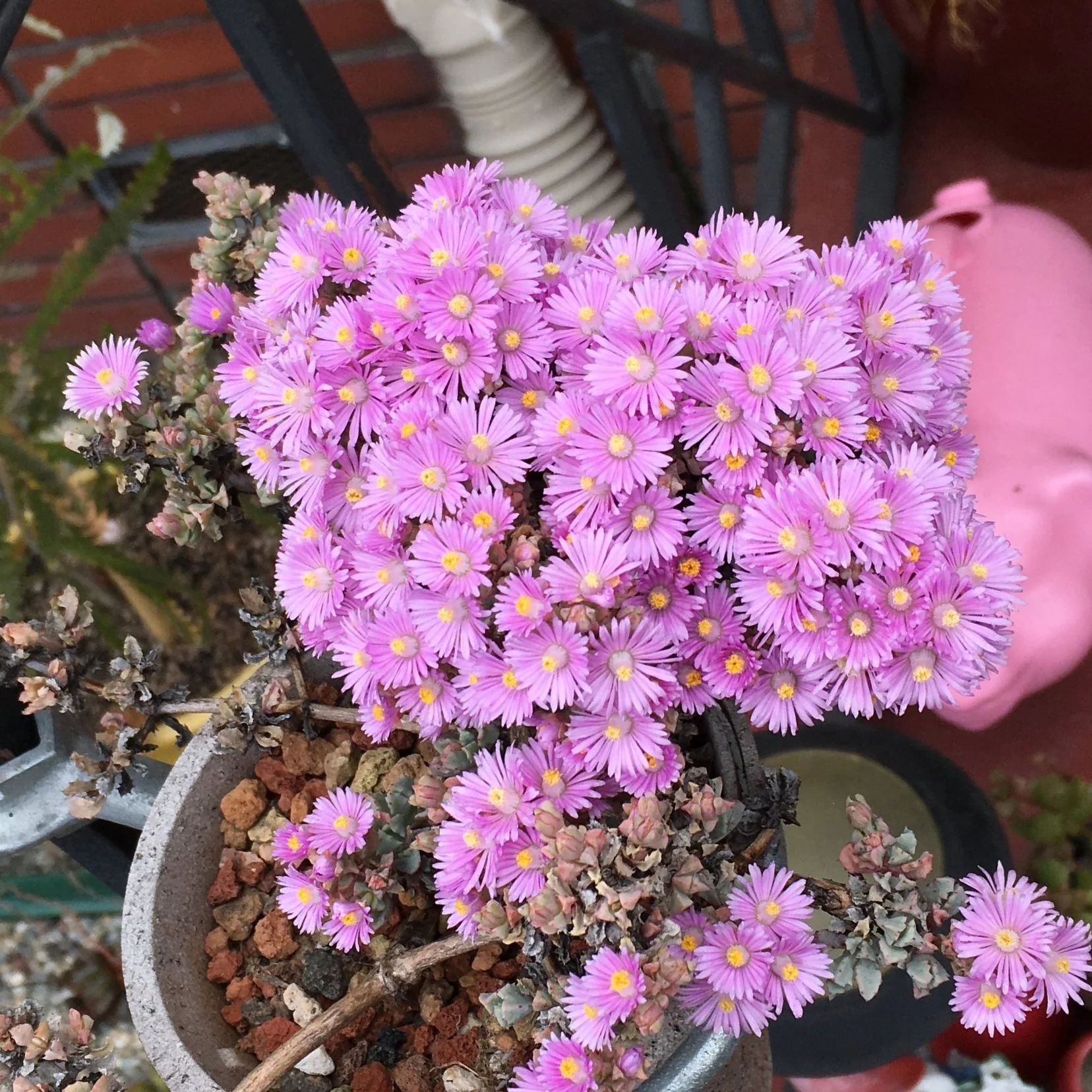
Electrocatalytic C–C Bond Cleavage Routes for Chemical Upcycling
The search for sustainable synthesis has provided insight into C–C bond formation during small molecule catalysis. In contrast, methods for breaking C–C bonds are important for industrial synthesis from crude oil. As a green alternative for top-down synthesis, we will study mild electrochemically driven C–C bond cleavage in hydrocarbons to mimic oil cracking and create future paths for upcycling difficult-to-transform plastics. We will investigate alternating potential electrochemistry for combining oxidative cleavage and reductive release of hydrocarbons and polymers at metal electrodes for green fuel and chemical synthesis that will create valuable carbon feedstocks. Additives like CO or CN– can also be introduced to intercept cracked alkyl intermediates and synthesize new bonds using the potential to control the polarity of adsorbates. This work will develop new techniques to study hydrocarbon-electrode interactions and provide fundamental insight into how dynamic applied potential methods can control adsorption, oxidation, reduction, and desorption steps for sustainable synthesis.
Doctoral Research - Hybrid Molecular Design Strategies for Electrosynthesis of Value-Added Products
Manipulating chemical transformations of CO2, O2, H2O, CH4, NO3–, and N2 is crucial for establishing sustainable energy cycles that can be driven using renewable electricity. Developing platforms that can activate these molecules is a continuous challenge and must be approached from many directions. As such, I took inspiration from homogeneous, heterogeneous, and biological catalysis to create hybrid platforms with selected benefits from each field, including atomically precise design, high surface area, and guided substrate delivery.

Postdoctoral Research - Molecular and Materials Solutions to Synthesize and Screen Millions of Nanoparticle Catalysts
The discovery of improved catalysts is central to fostering a greener chemical industry, but for inorganic nanomaterials, discovery is slowed by the relatively difficult synthetic control compared to molecular synthesis and the enormous materials design space encompassing composition, size, shape, morphology, and support, among others. Variation of composition alone yields a near infinite number of conceivable combinations. To address this, we created a platform for synthesizing and screening nanoparticle catalysts at unprecedented speeds.